Research
Why structural anisotropy in soft materials?
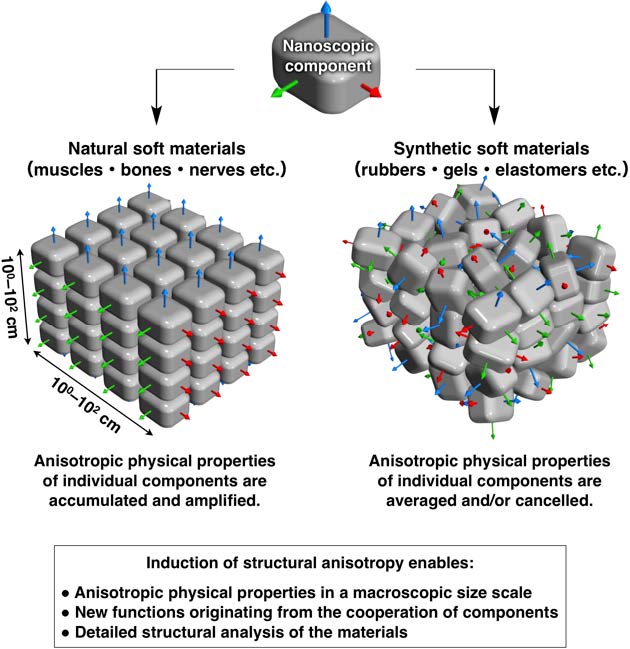
Owing to their lightweight, flexibility, and biocompatibility, soft materials have attracted increasing attention from various fields. They are intrinsically similar to living tissues and therefore promising as the components artificial organs. However, in terms of structure, soft materials are substantially different from biological systems. Synthetic soft materials are composed of “isotropic” polymer networks, whereas most biological systems adopt “anisotropic” structures with hierarchically integrated building units up to a macroscopic length scale, as represented by muscle, blood vessels, articular cartilage, and iridophores. Such anisotropic structures play an essential role in biological systems, exhibiting specific functions, including actuation, mass transport, surface lubrication, anisotropic mechanical properties, and visual sensing. In this context, anisotropic soft materials would provide an entry point for exploring biomimetic applications of soft materials.
The importance of orientation control in soft materials has long been pointed out, and many studies have already been conducted. However, most previous studies have limited their scopes only to confirm the anisotropy in physical properties, while attractive functions of oriented materials have rarely been explored. One of the reasons why this field has not made great progress is the difficulty in obtaining ideal materials. Although the orientation control within thin fibers and films is sometimes possible, it is extremely difficult to uniformly and continuously construct a highly oriented structure over a bulk material in a large area and a large thickness. Meanwhile, for physical characteristics, optical characteristics, deformation characteristics, material characteristics, etc.), a sample with a size of at least several mm is required.
As described in the following sections, we have established the method to introduce structural anisotropy into synthetic soft materials, for making them as close to living organisms as possible. In fact, the induction of structural anisotropy in soft materials enables the followings:
- Anisotropic physical properties in a macroscopic size scale.
- New functions originating from the cooperation of components.
- Detailed structural analysis of the materials.
Recent developments in materials with macroscopic anisotropy, including our research, are summarized in the following review articles. These review articles proved tables that categorize past researches by material, orientation method, and function, which would be convenient for those who want to learn this field quickly.
References
Adv. Mater. 2017, 29, 1605974.
Angew. Chem. Int. Ed. 2018, 57, 2532–2543.
Langmuir 2020, 36, 11702–11731.
How to synthesize anisotropic soft materials?
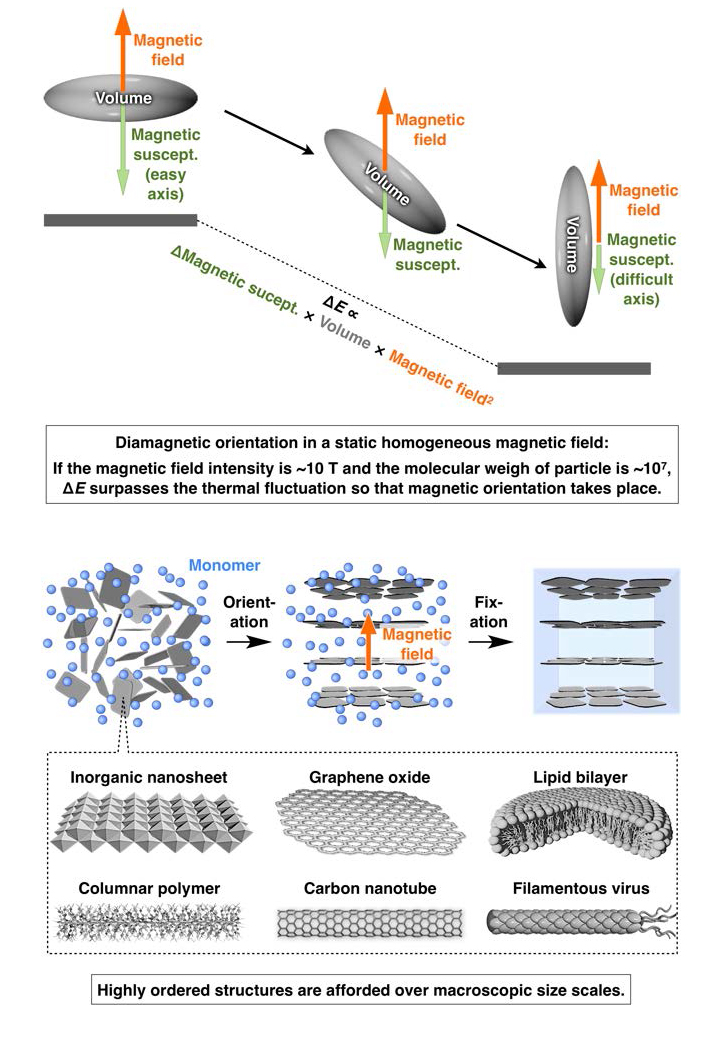
Then, how can we synthesize a sample of anisotropic soft material that has a size and orientation that can withstand the evaluation of physical properties? For this aim, we are focusing on the magnetic field. Although various fields are available for inducing the structural anisotropy of materials, the magnetic field is the only field that can be applied non-contact and non-destructively. In addition, unlike other external fields, the magnetic field penetrates homogeneously into the interior regardless of the thickness of the sample. If we need a uniformly oriented soft material with a thickness of a few millimeters or more, the magnetic field orientation is the only option.
The drawback of magnetic fields is that they cannot act strongly on “diamagnetic substances” that are the majority of materials. The orientation energy of diamagnetic particles in a static homogeneous magnetic field is promotional to Δ(magnetic susceptibility) × (particle volume) × (magnetic intensity)2.Since diamagnetic materials generally have extremely low magnetic sensitivity, it must be said that they are not suitable for magnetic field orientation. However, if we look at the above equation, we can see that efficient orientation occurs even in diamagnetic materials by using a strong magnetic field of more than 10 T and a huge nanostructure with a molecular weight of more than 107.
Since the rapid progress of superconducting engineering and nanomaterial science has made it easier to prepare the above two conditions (strong magnetic field and large nanostructures), the magnetic field orientation should be regarded as one of the most reliable methods for inducing structural anisotropy of materials. Indeed, we have developed macroscopically anisotropic soft materials, in which highly ordered structures (order parameter = 0.95–0.99) extend over macroscopic size scales (100–102 mm).
So far, we have challenged the orientation of various 1D and 2D nanostructures such as inorganic nanosheet, graphene oxide, lipid bilayer, columnar polymer, carbon nanotube, and filamentous virus. These anisotropic soft materials exhibit amazing physical properties and functions that are reminiscent of living organisms. Please see the respective sections for more details on the individual examples.
Hydrogel with mechanical anisotropy similar to articular cartilage
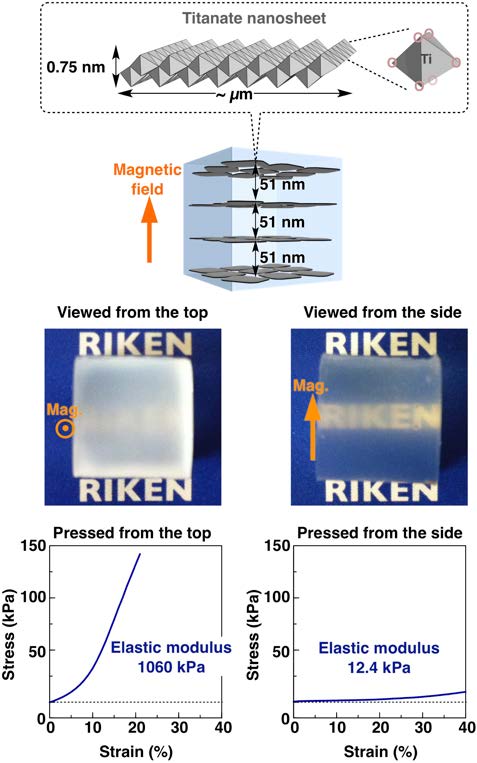
The titanate nanosheet, developed by Sasaki et al.in 1996, is a 2D crystal in which a large number of TiO6 octahedrons are spread in a single layer, and has an extremely anisotropic shape with a width of ~10 μm and a thickness of ~1 nm. It has a high density of negative charges and is stably dispersed in water due to mutual electrostatic repulsion. We have found that this nanosheet exhibits interesting magnetic field orientation behavior. When a magnetic field is applied to the aqueous dispersion of titanate nanosheets, the nanosheets are oriented perpendicular to the magnetic field, so that all nanosheets in the system are oriented in the same direction. As clarified by 2D SAXS measurement, the nanosheets are not only unidirectionally oriented, but also have a highly ordered lamellar structure like a “giant single crystal”, in which the nanosheets are arranged at long and regular periodicity of 51 nm. A huge and anisotropic electrostatic repulsive force and van der Waals attractive force act between adjacent nanosheets, and the distance between the nanosheets is defined by the balance between the two forces.
The magnetically oriented structure is relaxed when the magnetic field is removed, but can be chemically fixed by polymerizing the coexisting acrylic monomers under the magnetic field. The resulting hydrogel exhibits significant mechanical anisotropy. Thus, the hydrogel easily deforms in the horizontal direction, while strongly resist to vertical forces. The origin of this particular mechanical properties is that nanosheets dislike approaching each other due to the electrostatic repulsion. When the electrostatic repulsion between nanosheets is maximized by thoroughly removing excess ions contained in the hydrogel and forming a gel network using a highly polar polymer, the mechanical anisotropy (= ratio of elastic modulus in the vertical direction and the horizontal direction) reaches up to ~80. By taking advantage of this remarkable mechanical anisotropy, excellent anti-vibration can also be achieved.
Research on composites of inorganic microparticles and organic polymers has a long history, but so far, the focus has only been on how to increase the attractive force between units. This study, which first demonstrated the usefulness of repulsive forces between units in controlling mechanical properties, sheds lights in the studies of composite materials.
Reference
Nature 2015, 517, 68–72.
Angew. Chem. Int. Ed. 2018, 57, 12508–12513.
Hydrogel that undergoes high-speed large deformation similar to muscle
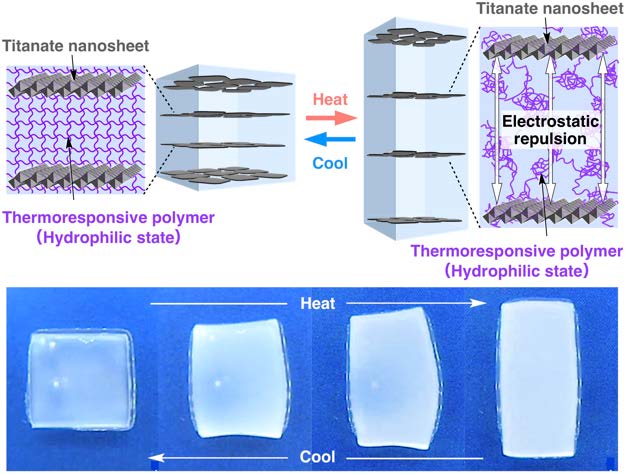
Using the same method as the mechanically anisotropic hydrogel in the previous section, we created a hydrogel in which titanate nanosheets were anisotropically embedded in a network of a thermoresponsive polymer. When this hydrogel is heated and cooled, the polymer is dehydrated and hydrated, so that the internal dielectric constant is also switched, thereby causing the increase or decrease of electrostatic repulsion between the nanosheets. Along with this, the entire hydrogel undergoes large deformation at high speed in the vertical direction of the nanosheets.
In fact, when this hydrogel is heated, it expands twice in the direction perpendicular to the nanosheets. At the same time, it contracts 0.8 times in the direction parallel to the nanosheets, and the volume of hydrogel is kept constant. When the hydrogel is cooled, the hydrogel returns to its original shape within 1 second. Since this transformation does not involve the transfer of water between the inside and outside of the hydrogel, it is extremely fast and can be repeated many times without deterioration. The deformation speed is the fastest among gel actuators. Synchrotron radiation 2D SAXS measurement proved that the inter-plane distance of the nanosheet and the length of the hydrogel keep a proportional relationship. Thus, the change in the nanostructure is directly reflected in the change in the macro shape, reminiscent of muscle movement that expands and contracts in response to structural changes in actin and myosin.
By cutting the hydrogel containing the diagonally oriented nanosheets into an L shape, it is possible to make an actuator that keeps walking in one direction. Before this work, for realizing unidirectional motion with a conventional actuator, a specially designed external environment such as a ratchet board or a directional external field is indispensable. On the other hand, this walking motion is the first example of creating a unidirectional motion from non- directional thermal energy using only the internal structure of the actuator.
Recently, we further improved our hydrogel actuator so that it can respond not only to heat but also light. When gold nanoparticles, which can convert light energy into heat energy, are doped in our hydrogel actuator, it deforms only in the light-irradiated part. Since the deformation can be programmed in a spatiotemporal and manner, so that more complicated movements become possible, such as the peristaltic movement of earthworms.
This hydrogel actuator surpasses conventional hydrogel actuators in quality and quantity of movement, and its development would be a major step closer to “artificial muscle”. In addition, the unprecedented operating principle can have a great impact on future related researches.
Reference
Nat. Mater. 2015, 14, 1002–1007.
Angew. Chem. Int. Ed. 2018, 57, 15772–15776.
Colloidal dispersion that quickly changes structure color
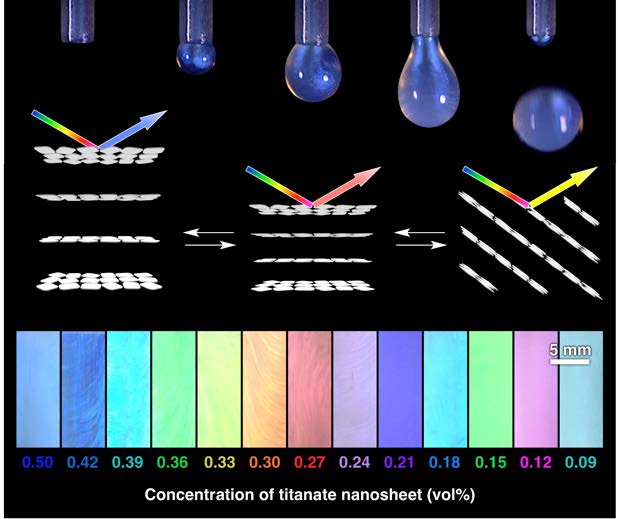
Photonic crystals have attracted many attentions as ultimate tools for controlling the properties of light, expected as the key for the next-generation photonics. As the name “crystal” implies, it must be a highly ordered structure with a period of several hundred nanometers, so it is usually composed of solid materials. The science of photonic crystals has long assumed that the size and orientation of the lattice cannot be changed. On the other hand, in nature, there are organisms (tropical fish, chameleons, etc.) that use dynamic photonic crystals consisting of fluids such as cytoplasm. They exhibit dynamic photonic functions that are impossible with artificial photonic crystals based on static solids, such as changing their body color at high speed in response to the environment. Then, what if we could construct a highly ordered dynamic photonic crystal mainly on a fluid such as water?
Recently, we serendipitously found that water (99%) containing an titanate (1%) can afford a high-quality photonic crystal. I succeeded in obtaining “crystals”. As the key of this finding, we noticed that the aqueous dispersion of titanate nanosheets contains excess ions that do not contribute for the colloidal stability of the nanosheets. These excess ions are added when the nanosheet are synthesized from the layered crystals, and the resultant nanosheet dispersion is usually used as it is. On the other hand, such excess ions are known to significantly shield the electrostatic repulsive force in the system. Therefore, when the nanosheet dispersion is thoroughly deionized, the dispersion showed a vivid structural color, where the electrostatic repulsion between the nanosheets is enhanced by ion removal, so that the distance between the sheets reaches the level of the visible light wavelength. Upon diluting the nanosheet dispersion, the sheet spacing can be expanded up to 675 nm.
Our dynamic photonic crystal, which is 99% or more made of water, can quickly change the angle and periodicity of the lamellar structure in response to the environment, so that its structural color can be changed accordingly. More recently, we established the method to tune the magnetic orientation mode of this photonic crystal by using photo- induced redox reaction of the nanosheets. This dynamic photonic crystal exhibits various functions that have never been expected in conventional static photonic crystals and therefore would bring innovation in the photonics field.
Reference
Nat. Commun. 2016, 7, 12559.
Angew. Chem. Int. Ed. 2018, 57, 12508–12513.
J. Am. Chem. Soc. 2018, 140, 16396–16401.
Porous polymer for arraying guest molecules along unidirectionally oriented helices
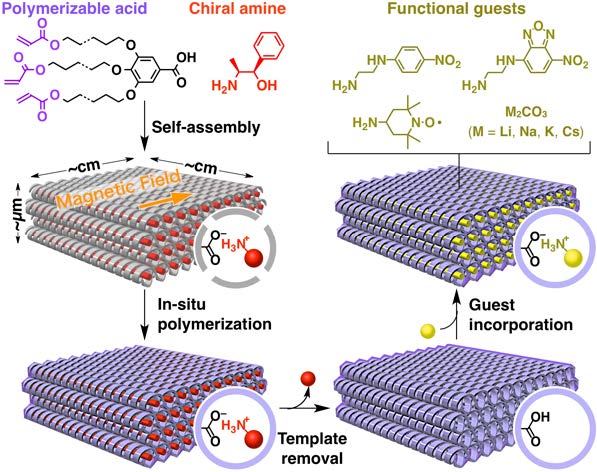
We have studied on covalently bonded porous materials prepared by in-situ polymerization of supramolecular liquid crystals composed of a salt of a carboxylic acid having a polymerization functional group and a chiral amine. The in-situ polymerization proceeds quantitatively while maintaining the liquid crystalline structure to afford a cross-linked polymer with highly ordered structure. Furthermore, the ordered structures maintained when the chiral amine is extracted by treating with an acid. The obtained crosslinked polymer memorizes the shape of the template amine even after the template is removed, and can be used for chiral recognition and chiral separation.
We recently found that the above supramolecular liquid crystal can be unidirectionally oriented under a strong magnetic field. Therefore, the fabrication of the liquid crystal under a 10 T magnetic field, in-situ polymerization of the acid unit, and subsequent amine removal afford a film of porous polymer with a covalent framework that is uniquely oriented over a large area. Although this material is a polymer linked by covalent bonds, it exhibits an X-ray diffraction pattern as if it were a single crystal, so detailed structural analysis can be performed by a method similar to single crystal X-ray structural analysis.
The resultant cross-linked polymer can encapsulate guest molecules, where the guest molecules are anisotropically oriented a large area. Interestingly, when a nonlinear optical dye was introduced as a guest molecule, the resulting oriented sample showed a nonlinear optical effect about 10 times stronger than the non-aligned sample prepared without applying a magnetic field. Since the pores in the cross-linked polymer helical and therefore intrinsically noncentrosymmetric, the dye can show nonlinear optical effects. Owing to the unidirectionally oriented structure over a region of several centimeters, the nonlinear optical signals emitted by the dye molecules become coherent, thereby showing enhanced intensity.
This porous polymer can encapsulate not only non-linear optical dyes but also various guest molecules such as those with fluorescence emission, those with stable radical sites, and alkali metals. It is expected to be applied as a versatile molecular grid that can arrange such functional guest molecules in a noncentrocymmetric arrangement over a macroscopic size scale, which is ideal for exhibiting nonlinear physical responses.
The scope of this project includes the development of other magnetically orientable 1D nanoobjects other than the above supramolecular columnar liquid crystal, such as supramolecular polymers and bottlebrush polymers.
Reference
Nat. Commun. 2015, 6, 8418.
Nat. Commun. 2020, 11, 1.
Angew. Chem. Int. Ed. 2021, 60, 1528–1534.